With precision tools, synthetic biology has enormous implications, offering hope against some of our major global challenges.
Available at supermarkets for a few bucks and existing since before recorded history, brewer’s yeast is too commonplace to excite most people. But University of California, Berkeley biochemical engineer Professor Jay Keasling is not most people.
Routinely used to make bread and ferment alcoholic beverages, saccharomyces cerevisiae has long been a dietary staple and has been studied extensively by Keasling and his peers as a model organism.
He has spearheaded high-impact research on the microbe, using gene editing to tweak its innards so it can digest sugar and excrete what eventually becomes antimalarial treatment – which could potentially save countless lives.
“More than 15 million people have gotten access to those treatments produced using the engineered yeast,” he told create, nominating the work as the most satisfying he has been involved in. S. cerevisiae and other microbes, through the application of synthetic biology, can be made to produce all kinds of useful things.
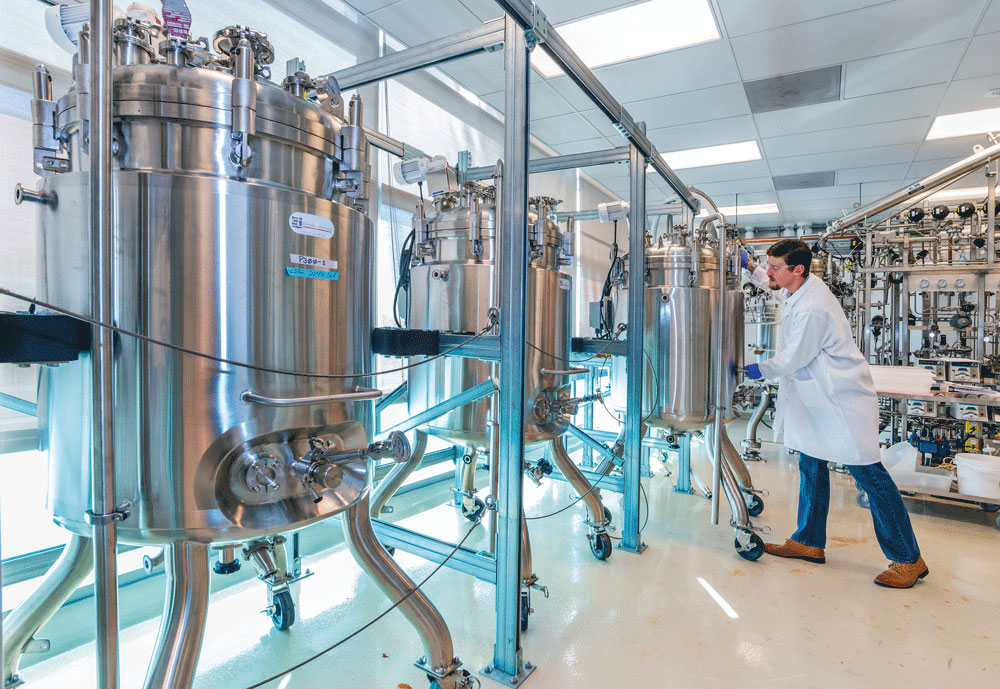
They’re like “little chemical factories”, Keasling said, and their metabolic pathways are able to be altered to serve numerous ends. In a world with an increasing strain being applied to its finite resources, manmade microbial strains could be crucial in solving many of our biggest problems.
One leading synthetic biologist, Harvard’s Pamela Silver, has suggested that biology, rather than anything else, is the technology of the 21st century. Does Keasling agree?
“Of course it’s the technology of the 21st century! Why not?,” he declared, almost sounding insulted by the question.
“It’s going to have enormous implications.”
Synthetic biology
The definition of synthetic biology – a mix of biology, computer science, engineering and other disciplines – is elusive and depends on who you ask.
“Genetic engineering on steroids” is one attempt, and Keasling has suggested it’s simply “engineering biology to do things it wouldn’t normally do.”
The title goes back to the mid-70s, around the time genetic engineering was emerging, though its principles began to be articulated early this century. Leaders such as Keasling, George Church and Drew Endy stressed the electronics engineering paradigm as a model. The ability to build circuits from standardised parts and devices, and consistent improvements in technology enabled by this, are cited. The goal was to borrow from the approach of planar process manufacturing.
Early problems addressed by the field have been in areas such as diagnosis, chemical manufacturing, and biofuels. Though synthetic biology hasn’t led to affordable, renewable fuel for all, the discipline has gotten much more sophisticated.
An iteration – in which new life is literally designed and created – is now only weeks in the making, where it might’ve formerly taken years. Biofoundries see life sketched out in CAD software, designs sent to a robot in another room to assemble, and machine learning applied to the results.
“It’s changed dramatically since I started my career,” Keasling said.
“When I started, the tools were so rudimentary and it took so long to get through the design, build, test cycle – it might change an entire PhD thesis to get around it.”
Machine learning is becoming “really hot”, and while it’s still early days, Keasling sees it as having huge potential in terms of learning from successes and failures. Are things getting nearer to the predictability levels in electronics engineering, though?
“It’s getting closer all the time but we’ve got a long way to go,” answers Keasling. “But that’s good. It keeps me in business.”
Fuels and the future
In July 2014 a Boeing 737 flew from Orlando to Sao Paolo on 10 per cent farnesane (produced using genetically engineered brewer’s yeast) blended with jet fuel. Biofuels aren’t competitive with low-margin diesel or petrol, but this is less the case for aero fuels.
“I think right now, because the price of oil is so low, it’s very hard for anything to compete with oil,” said Keasling, who is also CEO of the US Department of Energy’s Joint BioEnergy Institute.
“We’ll have the best chance of competing with bio fuels within the area of jet fuel because it’s a higher priced thing.”
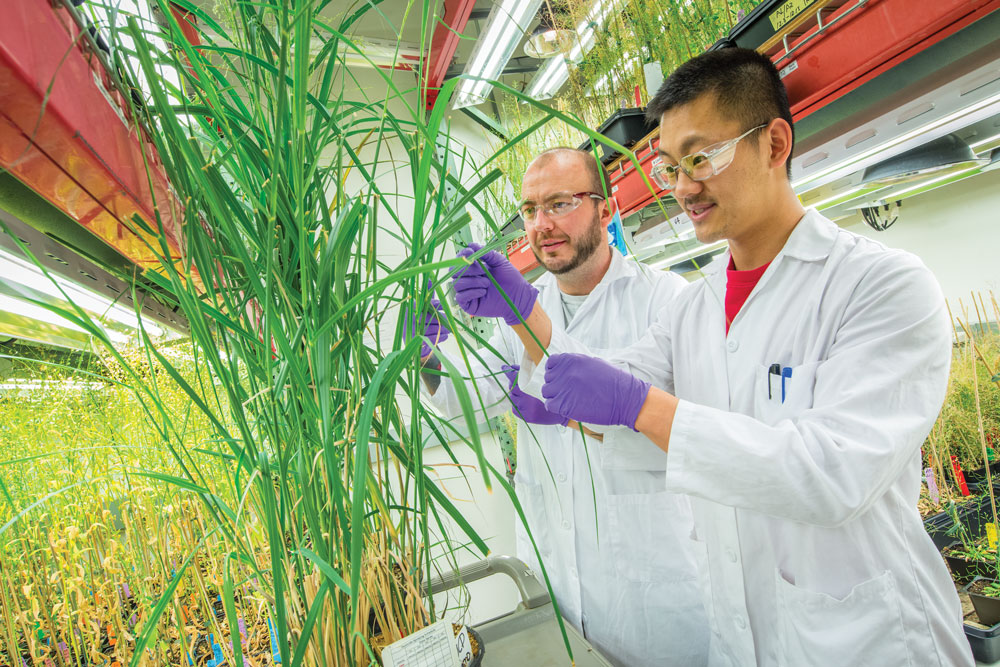
Keasling’s work concerns drop-in hydrocarbon biofuels, rather than ethanol, which he likes to say is good “for drinking, not for driving”. Ethanol cannot use the existing infrastructure used by the fossil fuel industry (such as pipelines and tanker trucks) or be substituted in vehicles.
Early synthetic biology companies such as LS9, Solazyme and Amyris (which Keasling co-founded) set out trying to produce sustainable fuels within their little chemical factories, but success was elusive. Several of them ended up changing course to produce higher-margin specialty chemicals for industries such as cosmetics or food manufacturing.
Amyris’s second project after antimalarial medicines was farnesene, sold under the name Biofene. The company describes this as a “renewable hydrocarbon building block”, made out of cane sugar by the reprogrammed yeast. Hydrogenated, it makes a diesel drop-in fuel (farnesane), and has been approved to be used as a blendstock in jet fuels.
The production cost of farnesene has come down considerably since the company began, from $US12 a litre at the beginning of 2013, to $US4 a litre later that year, and then $US1.75 in September last year. Though production is getting cheaper, for a company like Amyris to be competitive with makers of fossil fuels, carbon penalties would be needed.
“If you burn petroleum-based fuels in your car or your plane or whatever, it goes into the atmosphere, nobody has to pay anything for that and it’s causing an environmental collapse,” Keasling said.
“If there was a real tax on carbon, Amyris’s diesel might be cost-competitive. So it’s kind of an unfair playing field.”
Despite his concerns about the sustainability of current approaches towards conventional fuels, the chemical engineer is optimistic about the future and about what can be accomplished with good old S. cerivisiae. At the mention of the subject, he confessed his excitement again, and reminded us that we’re just getting started when it comes to exploiting the ancient microbe. With ever-more powerful tools and the right minds on the task, things just might be okay.
“I think there’s huge potential in terms of engineering yeast and we’re just seeing the potential right now, but I think there’s so much more to do,” Keasling said.
“I’d like to see us turn brewer’s yeast into something known to be a hydrocarbon overproducer.”